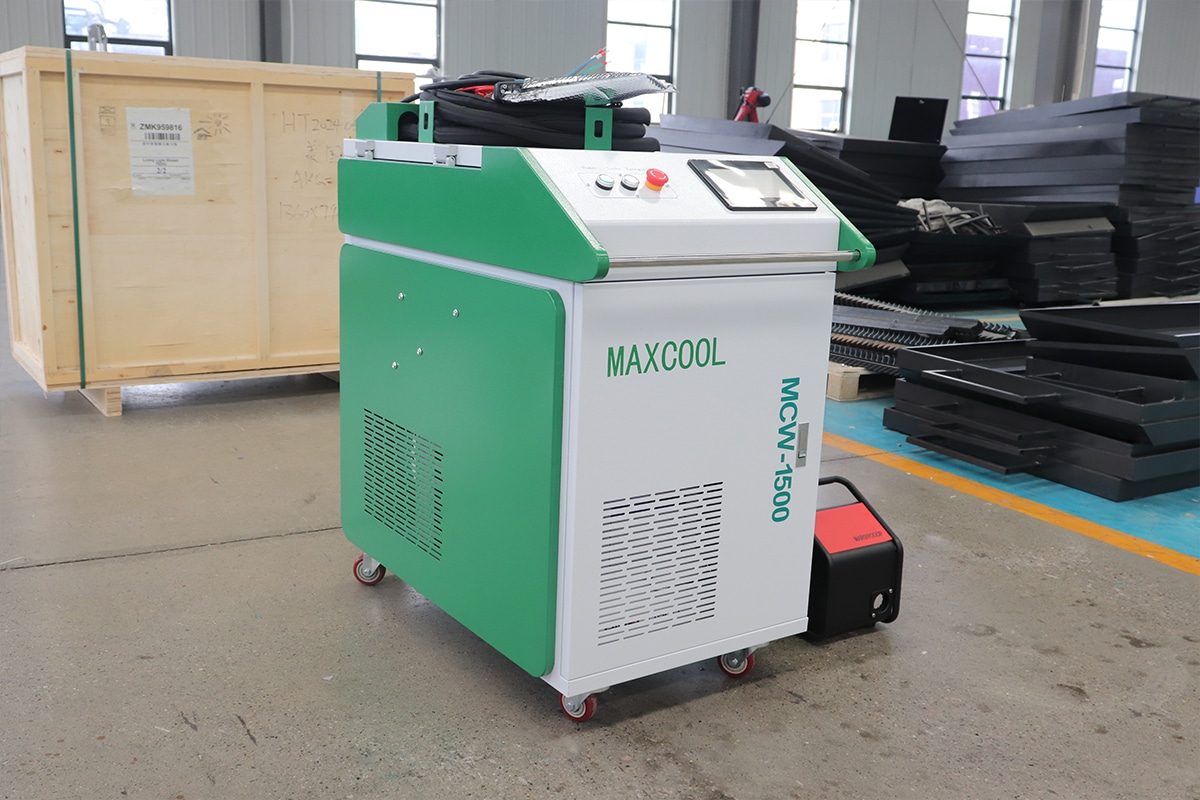
Fiber Laser Welding: A Brief Overview
The key advantages of fiber laser welding over traditional methods include:
- Energy Efficiency: Fiber lasers offer an energy conversion efficiency of up to 35%.
- High-Precision Welding: The small beam diameter of fiber lasers allows for precise control, making them ideal for micro-welding and automated welding applications.
- Material Flexibility: Fiber lasers are highly effective for a wide range of materials, including high-reflective metals such as aluminum, brass, and copper, although these materials can present challenges in terms of laser reflectivity.
Factors Affecting the Maximum Welding Thickness
Laser Power and Its Effect on Thickness
Laser power is the most critical determinant of the maximum welding thickness. In fiber laser welding, power is measured in watts (W), and it represents the amount of energy the laser can deliver to the material being welded.
- 1kW Laser (1000W): Fiber lasers operating at 1kW are generally used for thin materials. The maximum thickness for mild steel is around 2 mm, while for stainless steel, it can reach 1.5-2 mm. For reflective metals like aluminum, the maximum thickness is limited to 1.5-2 mm due to aluminum’s high reflectivity and thermal conductivity.
- 5kW Laser (1500W): With a 1.5kW laser, fiber welding can handle 3-4 mm of steel and up to 3 mm of aluminum. This power level is common in industries like automotive manufacturing, where precise welds are needed on parts of moderate thickness.
- 2kW Laser (2000W): At this power level, fiber lasers can weld materials up to 3-4 mm thick. For aluminum, this extends to around 3 mm, making it suitable for aerospace components and high-strength fabrications.
- 3kW Laser (3000W): This is the upper limit for fiber laser welding in this power range, capable of welding up to 6 mm in mild steel and 4 mm in aluminum. It’s used in heavy-duty applications such as shipbuilding, structural steel fabrication, and industrial machinery.
Material Properties
Different metals respond to laser energy in varying ways due to their thermal conductivity, reflectivity, and melting points. These factors significantly impact the maximum welding thickness achievable with fiber lasers.
- Mild Steel: Mild steel is one of the most laser-friendly metals due to its ability to absorb laser energy efficiently. The maximum thickness achievable with a 1kW laser is around 3 mm, while 3kW lasers can weld up to 6 mm thick mild steel. Steel’s relatively low thermal conductivity ensures that the heat generated by the laser is concentrated at the weld site, allowing for deep penetration.
- Stainless Steel: Stainless steel has higher thermal conductivity than mild steel, meaning it requires more energy to achieve the same penetration. 1kW fiber lasers can weld up to 1.5-2 mm of stainless steel, while 3kW lasers can weld up to 5-6 mm. Stainless steel’s resistance to corrosion makes it ideal for applications such as medical devices, pharmaceutical equipment, and food processing machinery.
- Aluminum: Aluminum poses unique challenges for laser welding due to its high reflectivity and thermal conductivity. These properties require higher laser power for deeper penetration. A 1kW laser can weld up to 2 mm of aluminum, while a 3kW laser can handle up to 4 mm. Given aluminum’s increasing use in lightweight structural components (especially in the automotive and aerospace sectors), fiber laser welding provides a viable solution for joining this material despite its challenges.
- Copper and Brass: Copper and brass have high reflectivity and thermal conductivity, which makes them more difficult to weld. However, with a 3kW laser, copper can be welded up to 1.5-2 mm thick, though reflective losses need to be carefully managed. Special techniques such as using green lasers (which are absorbed more efficiently by copper) or adaptive beam shaping can enhance welding performance for these materials.
Material Absorption and Reflectivity
Welding Speed and Heat Input
The welding speed, or the rate at which the laser beam moves across the material, is another crucial factor in determining the maximum welding thickness. Slower welding speeds allow the laser to deliver more energy into the material, leading to deeper penetration. However, slower speeds also increase the risk of thermal distortion and burn-through, especially when welding thin materials.
- Welding Speed vs. Thickness: For thick materials, reducing the welding speed can enable the laser to penetrate deeper. For instance, when welding 8 mm steel with a 2kW laser, a slower speed ensures sufficient energy for full penetration. Conversely, for thinner materials, faster welding speeds can be used to prevent overheating and ensure clean welds without compromising structural integrity.
- Heat Input: The amount of heat delivered to the material during welding must be carefully controlled. Excessive heat can cause issues such as cracking or distortion in materials like stainless steel and aluminum, especially when welding thicker sections. Adjusting the welding speed, laser pulse frequency, and power density can help optimize the heat input.
Joint Configuration and Design
The joint configuration—how the pieces of material are arranged for welding—directly impacts the maximum thickness that can be welded. Different joint types require different approaches to ensure full penetration and strong welds.
- Butt Joints: Butt joints allow for deep penetration, as the laser can focus directly on the seam between two pieces of material. For 3kW lasers, this configuration can weld up to 6 mm of steel or 4 mm of aluminum. Butt joints are commonly used in pipe welding, structural applications, and automotive panels.
- Lap Joints: Lap joints, where two pieces of material overlap, require more laser power for the same thickness because the laser must penetrate both layers. The maximum thickness for lap joints is typically 30-40% less than that for butt joints. For example, a 3kW laser might weld 3-4 mm in lap joints compared to 6 mm in butt joints.
- Edge Joints: Edge joints are easier to weld with less power because the laser can concentrate on a smaller area. This configuration is useful for sheet metal applications where thin materials need to be welded without excessive heat input.
- Fillet Joints: Fillet joints, commonly used in corner welding, can be more challenging to achieve full penetration because the laser must weld across two surfaces. Fillet joints are frequently used in structural welds and automotive chassis fabrication.
Beam Quality and Focusing
The quality of the laser beam—often measured by the M² factor—is critical for achieving the maximum thickness. The M² factor indicates how well the laser beam can be focused into a small spot, which directly impacts the welding depth and quality.
- Beam Quality: A lower M² value indicates a higher-quality beam with better-focusing capability, enabling deeper penetration. For thicker materials, maintaining excellent beam quality ensures the laser can concentrate energy at the weld site without scattering or reducing intensity.
- Focal Length: The focal length of the laser’s lens affects the penetration depth. A shorter focal length allows the beam to concentrate on a smaller area, resulting in deeper penetration, especially in high-density materials like steel. For welding thick materials, optimizing the focal length is crucial to achieving the maximum thickness without sacrificing weld quality.
- Focus Point Management: Maintaining the correct focus point ensures that the laser delivers maximum energy to the material. Automatic focusing systems are often used in industrial laser welding machines to maintain a consistent focus, especially when welding materials of varying thicknesses or complex geometries.
Challenges in Welding Thick Materials
Thermal Distortion and Warping
When welding thick materials, the amount of heat input increases significantly, leading to the risk of thermal distortion. This occurs when the material expands unevenly due to localized heating, causing the welded section to warp or bend.
- Aluminum and Stainless Steel are particularly prone to thermal distortion due to their high thermal conductivity. Excessive heat can cause these materials to lose their structural integrity, resulting in weakened welds or misalignment of parts.
Solution: To minimize thermal distortion, it is essential to:
- Reduce heat input by optimizing the welding speed and power settings.
- Use pulsed laser modes, which deliver energy in short bursts, allowing the material to cool between pulses.
- Incorporate fixturing techniques to hold the material in place during welding.
Porosity and Cracking
Porosity occurs when gas is trapped within the weld pool, resulting in voids that weaken the weld. Cracking may occur due to thermal stresses, particularly in thick materials where cooling rates can vary significantly between the surface and interior of the weld.</br>
Solution: Preventing porosity and cracking requires careful control of the welding environment:
- Use shielding gases (e.g., argon or helium) to protect the weld from atmospheric contamination.
- Optimize the welding speed to ensure a stable weld pool that cools evenly.
- For highly reflective materials like aluminum, using green lasers or adaptive beam shaping techniques can reduce reflectivity and improve weld quality.
Inconsistent Penetration
Best Practices for Welding Thick Materials with Fiber Lasers
To maximize the potential of fiber laser welding for thick materials, manufacturers and welders must follow several best practices:
- Optimize Power and Speed Settings: The right combination of power and speed is critical for achieving full penetration without overheating the material. For example, when welding 6mm thick steel, a 3kW laser may require a slower welding speed to ensure adequate heat input.
- Ensure Proper Beam Focus: Regularly adjust the focal length and monitor the focus point to maintain a sharp, concentrated beam. Automatic focusing systems are highly recommended for thick material welding, where maintaining a consistent focal point is essential for deep penetration.
- Use Proper Shielding Gas: The choice of shielding gas depends on the material being welded. Argon is commonly used for steel, while helium may be preferred for aluminum to prevent oxidation and improve weld quality.
- Monitor Joint Design: Select the appropriate joint design for the application. Butt joints are the most effective for welding thick materials, but lap joints or fillet joints may require more power or slower speeds.
- Control Heat Input: Avoid excessive heat input by adjusting the welding parameters and using pulsed laser modes. This helps reduce the risk of thermal distortion and ensures consistent penetration across the entire weld.
Applications of Fiber Laser Welding in Various Industries
Automotive Industry
In the automotive industry, fiber laser welding is used to join steel and aluminum components in vehicle frames, body panels, and exhaust systems. The ability to weld thick materials while maintaining precision ensures the structural integrity of lightweight vehicles.
- Example: Automakers are using fiber lasers to weld aluminum body panels for electric vehicles (EVs) to reduce weight without compromising strength.
Aerospace Industry
The aerospace industry requires welding techniques that can join high-strength alloys while minimizing weight. Fiber lasers are used to weld thick materials like titanium and aluminum in aircraft structures, engines, and fuselage components.
- Example: 3kW fiber lasers are used to weld up to 3 mm thick titanium in aircraft frames, ensuring high-strength joints that can withstand extreme conditions.
Shipbuilding
Shipbuilding involves welding thick steel plates to form ship hulls and structural components. Fiber laser welding allows for deep penetration with minimal distortion, making it an ideal solution for large-scale metal fabrication.
- Example: Fiber lasers are used to weld 5-6 mm thick steel plates in the construction of ship hulls, providing strong, watertight joints.
Heavy Equipment Manufacturing
Manufacturers of heavy equipment, such as construction machinery, agricultural equipment, and mining machinery, rely on fiber laser welding to join thick metal parts that require deep penetration and high structural integrity.
- Example: 3kW fiber lasers are commonly used to weld steel components for bulldozers, excavators, and cranes, where weld strength and durability are paramount.